CRISPR-Cas9: Revolutionizing Gene Editing in Biomedical Research
Abstract:
Biosimilars, a burgeoning field within the pharmaceutical industry, hold immense promise in revolutionizing the accessibility and affordability of biologic medicines. This article explores the complexities of biosimilars, including their development process, regulatory challenges, economic impact, and future prospects. By delving into these intricacies, we aim to provide valuable insights for commercial biopharmaceutical and academia researchers navigating the dynamic landscape of biosimilars.
Introduction:
In the realm of biomedical research and genetic engineering, CRISPR-Cas9 has emerged as a groundbreaking tool, transforming the capabilities of scientists to edit genomes with precision and efficiency. This innovative technology, known for its potential to modify gene expression and create genetically modified organisms (GMOs), has revolutionized the approach towards understanding and curing genetic diseases. By leveraging a simple yet powerful system of guide RNA and the Cas9 enzyme, CRISPR-Cas9 has not only made gene editing more accessible but has also opened new frontiers in functional genomics, showcasing its transformative potential across various fields of science.
The article unfolds the intricate workings of CRISPR-Cas9, starting from the basics of its mechanism to its profound applications in biomedical research. It will delve into the critical role of guide RNA in directing the Cas9 enzyme to specific genomic locations, ensuring high precision in gene editing endeavors. By exploring a spectrum of applications, from disease treatment and gene therapy to its implications in the creation of disease models, this piece aims to provide a comprehensive understanding of CRISPR technology. Furthermore, it addresses the challenges and ethical considerations inherent in its use, reflecting on the ongoing debate surrounding CRISPR gene editing, its safety, and its future prospects. Through this exploration, readers will gain insights into the remarkable versatility and efficiency of CRISPR-Cas9, which stands not only as a tool in functional genomics but also as a beacon of hope for innovative treatments and advancements in the field of genomics and beyond.
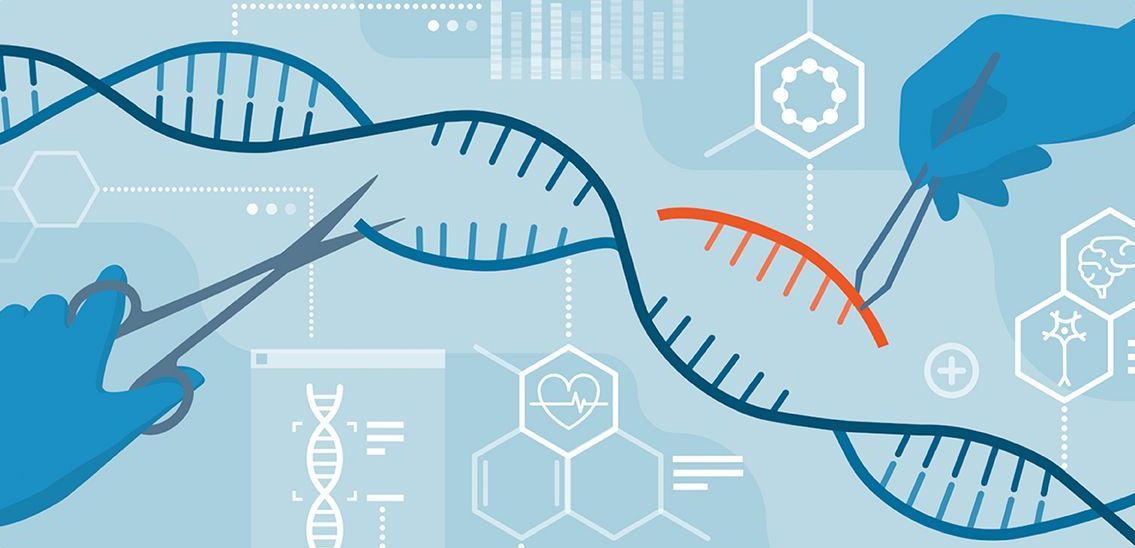
Overview of CRISPR-Cas9 Technology
Historical Discovery and Development
CRISPR-Cas9, standing for Clustered Regularly Interspaced Short Palindromic Repeats, was initially discovered as part of a bacterial adaptive immune system, protecting against foreign genetic elements. The foundational work began with the identification of unusual repetitive DNA sequences in Escherichia coli in 1987 by Ishino and his team, though the biological function of these sequences was not understood at the time. The term CRISPR was coined by Francisco Mojica in the 1990s, who recognized these sequences in archaea and later hypothesized their role in microbial immunity.
The transformative potential of CRISPR was realized when Emmanuelle Charpentier and Jennifer Doudna engineered CRISPR to function as a gene-editing tool in 2012, demonstrating its ability to cut DNA at specific locations. This discovery was pivotal, as it allowed for precise modifications of the genome by guiding the Cas9 enzyme to specific DNA sequences.
Mechanism of Action
The CRISPR-Cas9 system functions through a simple yet sophisticated mechanism involving two key components: the Cas9 protein and a piece of guide RNA (gRNA). The Cas9 protein acts as a molecular scissor that makes cuts in the DNA strand. The guide RNA, approximately 20 base pairs long, binds to a complementary DNA sequence adjacent to a Protospacer Adjacent Motif (PAM), which is crucial for the Cas protein to recognize the target DNA site.
Upon binding, the Cas9-gRNA complex unwinds the DNA helix and positions the DNA within the enzyme's active site to introduce a double-stranded break, which can then be repaired by the cell's natural repair machinery, thus allowing for gene editing. This process is highly efficient and precise, making CRISPR-Cas9 a powerful tool for genetic engineering.
Comparison with Other Genome Editing Tools
Prior to the advent of CRISPR-Cas9, the main tools for genome editing were Zinc Finger Nucleases (ZFNs) and Transcription Activator-Like Effector Nucleases (TALENs). These tools also utilized engineered nucleases to make targeted cuts in DNA; however, they were limited by the complexity and cost of protein engineering required to generate new sequence-specific nucleases for each target site.
CRISPR-Cas9 has several advantages over these earlier methods. It is significantly easier to design and implement due to the simplicity of programming the guide RNA. Additionally, CRISPR-Cas9 can target multiple genes simultaneously, a process that is much more cumbersome with ZFNs and TALENs. Furthermore, the efficiency and cost-effectiveness of CRISPR-Cas9 make it accessible for a broader range of scientific applications, from basic research to therapeutic development.
This overview highlights the groundbreaking nature of CRISPR-Cas9 technology, which has revolutionized the field of genetic editing by providing a simpler, more efficient, and versatile tool compared to its predecessors.
Overview of CRISPR-Cas9 Technology
In the CRISPR-Cas9 system, the guide RNA (sgRNA) plays a pivotal role in the gene-editing process. It is essentially the component that ensures precision, guiding the Cas9 enzyme to the correct part of the genome for editing. This section delves into the function and significance of sgRNA, as well as how it is designed and customized for precise targeting.
Function and Significance
The sgRNA is a fusion of CRISPR RNA (crRNA) and trans-activating CRISPR RNA (tracrRNA), combining into a single guide structure that is simpler and more efficient for mammalian genome editing. The crRNA component of sgRNA, typically 18–20 base pairs in length, is crucial as it specifies the target DNA sequence by pairing with it. This pairing is essential for the Cas9 enzyme to recognize and cleave the target DNA at the desired location. The sgRNA's design, particularly the gRNA domain, is critical for the efficacy and specificity of the genome-editing activities performed by Cas9. This has led to the development of multiple bioinformatics tools aimed at the rational design of sgRNAs, focusing on both targeting specificity and potency [17].
Experimental analyses have highlighted the potential for Cas9-based genome editing to result in off-target effects, underscoring the importance of designing sgRNAs with high specificity to minimize unintended genomic modifications. Furthermore, the efficacy of individual sgRNAs can vary significantly, making the design of potent sgRNAs a priority for efficient genome editing and resource utilization.
.
Design and Customization for Precise Targeting
Designing an sgRNA involves selecting a sequence that is complementary to the target gene sequence while ensuring the presence of a Protospacer Adjacent Motif (PAM) at the 3' end of the target DNA sequence. The PAM sequence is crucial for Cas9 cleavage but is not included in the sgRNA sequence. The targeting sequence, which lies upstream of the PAM, is typically 20 nucleotides long and directs Cas9 to cleave approximately three bases upstream of the PAM.
Online tools and algorithms play a significant role in identifying potential PAM sequences and predicting off-target effects, aiding researchers in selecting the most specific crRNA for their applications. Additional design considerations, such as the inclusion of specific nucleotides at certain positions within the sgRNA, have been identified through empirical research to enhance sgRNA efficiency. Despite careful design, sgRNA specificity and activity can be unpredictable, prompting the recommendation to design and test multiple sgRNAs for a given target gene.
The process of synthesizing sgRNAs involves generating a template DNA containing the sgRNA-encoding sequence, followed by in vitro transcription to produce sgRNAs that can be purified and tested for efficiency. This meticulous design and customization process is essential for achieving precise genome editing, minimizing off-target effects, and maximizing the potential of CRISPR-Cas9 technology in research and therapeutic applications.
Applications of CRISPR-Cas9 in Biomedical Research
Genetic Disease Treatment Advancements
CRISPR-Cas9 technology has significantly advanced the treatment of genetic diseases by enabling precise modifications of genetic material. Monogenic diseases, which are linked to over 75,000 genetic variants, affect a large population, with many conditions still lacking effective treatments. CRISPR-Cas tools are extensively used to correct genetic variants, offering hope for treating a wide array of human genetic diseases. Notable applications include treatments for inherited blood disorders like sickle cell disease, β-thalassemia, and hemophilia , eye diseases such as Leber congenital amaurosis and inherited retinal degeneration, and muscular genetic diseases like Duchenne muscular dystrophy. Additionally, CRISPR has been applied to genetic liver diseases, congenital genetic lung diseases including cystic fibrosis, neurological disorders, and genetic deafness.
Innovative studies illustrate the potential of CRISPR-Cas9 in treating genetic disorders effectively. For instance, a study by Wu et al. demonstrated the use of CRISPR/Cas9 to treat cataracts in a mouse model, resulting in successful gene repairs in several mice. Similarly, Schwank et al. utilized CRISPR technology to correct mutations in intestinal stem cells from cystic fibrosis patients, showcasing the practical implications of CRISPR in gene therapy.
Potential in Infectious Disease Management
CRISPR-Cas9 has also emerged as a powerful tool in managing infectious diseases. Its application spans from treating viral infections such as HIV and hepatitis to tackling resistant bacterial and fungal infections. Notably, CRISPR-Cas9 has been employed to inhibit multiple steps of HIV-1 infection effectively. The technology's adaptability extends to other viral infections, where it has shown potential in providing protective immunity against viruses.
The versatility of CRISPR is further highlighted in its use in developing rapid, low-cost diagnostic systems for infectious diseases. For example, the Cas13a protein has been utilized in the Specific High Sensitivity Enzymatic Reporter UnLOCKing (SHERLOCK) system, enhancing the detection of RNA viruses like SARS-CoV-2. This system represents a significant advancement in the rapid diagnosis and management of viral outbreaks.
Furthermore, CRISPR-based strategies are being developed to reprogram human B cells to produce neutralizing antibodies, offering a novel approach to treat and manage infectious diseases effectively. These applications underscore the potential of CRISPR technology not only in treating but also in preventing future global pandemics through improved diagnostics and vaccine research.
CRISPR-Cas9's role in biomedical research continues to expand, addressing complex challenges in genetics and infectious diseases. Its ability to edit genes with high precision makes it an invaluable tool in the medical field, promising revolutionary advances in treatment and disease management.
Challenges and Ethical Considerations
Despite the significant advancements and promising applications of CRISPR-Cas9 technology in biomedical research, there are notable challenges and ethical considerations that need to be addressed. These concerns span from technical hurdles in delivery systems to the broader ethical debates and regulatory landscape surrounding gene editing.
Technical Hurdles in Delivery Systems
One of the primary technical challenges in the application of CRISPR-Cas9 is the efficient delivery of its components to target cells in vivo. The use of viral vectors, such as adeno-associated viruses (AAVs), has been common due to their high transduction efficiency and relatively lower immunogenicity.
However, concerns regarding safety, including the risk of insertional mutagenesis and carcinogenesis, have been raised. Non-viral delivery systems, such as nanoparticles, offer advantages including reduced off-target effects and minimal immunogenicity. Despite these benefits, challenges such as lower delivery efficiency to non-liver tissues remain a significant hurdle for the therapeutic application of nanoparticle-mediated CRISPR/Cas gene editing systems.
The Cas9 protein, derived from bacteria like Streptococcus pyogenes, poses an additional challenge due to its recognition by the human immune system as a foreign antigen. This immune response can lead to the rapid degradation of the Cas9 protein, hindering its gene-editing function. Furthermore, selecting an appropriate delivery technique that ensures safe and precise targeting of the CRISPR system to the desired site, especially in vivo, is a critical consideration.
Limitations of Guide RNA and Off-Target Effects
The guide RNA (gRNA) is a crucial component of the CRISPR-Cas9 system, directing the Cas9 enzyme to specific genomic sequences for editing. However, the design and efficacy of gRNA can be challenging. The specificity of gRNA is paramount to avoid off-target effects, which are unintended modifications at non-target sites in the genome. Off-target mutations can have unpredictable consequences, potentially causing harmful effects or reducing the efficacy of the intended gene edit. Researchers continually work on improving gRNA design algorithms and developing methods to enhance target specificity, but achieving perfect accuracy remains an elusive goal.
Alternative CRISPR Systems: Cas12 and Cas13
To address some of the limitations associated with CRISPR-Cas9, alternative CRISPR systems like Cas12 and Cas13 have been developed. Cas12, also known as Cpf1, offers a different mechanism of DNA cutting that can enhance targeting specificity and reduce off-target effects. Cas12 recognizes a different protospacer adjacent motif (PAM) sequence than Cas9, expanding the range of possible target sites within the genome. Cas13, on the other hand, targets RNA instead of DNA, providing a unique tool for regulating gene expression and potentially treating RNA-based diseases. Cas13's RNA-targeting capability can be particularly useful for transient gene expression modifications without permanently altering the genome.
Ethical Considerations and Regulatory Landscape
Moreover, the ethical considerations surrounding CRISPR-Cas9 cannot be overlooked. The potential for germline editing, which involves making changes that can be passed down to future generations, raises profound ethical questions about consent, the nature of human enhancement, and the potential for unintended long-term consequences. Regulatory frameworks worldwide are grappling with how to balance the promise of CRISPR technology with these ethical concerns. As the technology continues to evolve, ongoing dialogue between scientists, ethicists, policymakers, and the public will be crucial in navigating these complex issues and ensuring responsible use of CRISPR-Cas9 in biomedical research and beyond.
By addressing these technological limitations and ethical considerations, the field can move towards safer and more effective applications of CRISPR technology, unlocking its full potential in advancing human health and disease treatment
Conclusion
Throughout this exploration, we have delved into the transformative impact of CRISPR-Cas9, uncovering its revolutionary applications in biomedical research and beyond. From its foundational mechanisms to its vast potential in disease treatment and gene therapy, this powerful gene-editing tool stands as a beacon of innovation, offering new avenues for addressing some of the most challenging medical and biological puzzles. The discussion on its ethical considerations and safety measures reflects the conscientious path that researchers and scientists are navigating to harness CRISPR-Cas9's capabilities responsibly and effectively.
As we look towards the future, the ongoing research and advancements within this field promise to broaden the horizon of what's possible with CRISPR-Cas9, potentially ushering in a new era of personalized medicine and targeted genetic therapies.
For researchers keen on leveraging the precision and versatility of CRISPR-Cas9, especially its guide RNA, in their experiments,
we invite you to discuss your questions or experimental needs with us.
As the field continues to evolve, the significance of keeping abreast with these advancements cannot be overstated, ensuring that the potential of CRISPR-Cas9 in revolutionizing biomedical research is fully realized in a manner that is safe, ethical, and impactful.
FAQs
Why is CRISPR-Cas9 considered a groundbreaking discovery?
CRISPR/Cas9 technology has significantly impacted genetic research, particularly in the study of human T cells. It enables immunologists to examine gene functions in a comprehensive manner, offering a higher translational potential in preclinical studies. This method is considered an advancement over traditional genetically modified animal models.
What role does CRISPR play in biomedical research?
In the realm of biomedical research, CRISPR is primarily utilized for genome editing. This innovative tool aids scientists in swiftly generating cell and animal models, which are crucial for advancing research into various diseases, including cancer and mental health disorders.
Why is CRISPR-Cas9 considered a groundbreaking discovery?
CRISPR-Cas9 is hailed as a groundbreaking discovery due to its ability to edit genes with high precision in human embryonic stem cells and its application in CRISPR-based single-cell molecular screens. Furthermore, it has been used in dropout screening to identify genes associated with lethal phenotypes, showcasing its potential for wide-scale genetic investigation.
What does CRISPR mean for biomedicine?
In biomedicine, CRISPR technology heralds a new era of personalized medicine. It enables the customization of treatments to fit a patient's unique genetic profile, leading to therapies that are more effective and specifically targeted to individual needs.
Explore kbDNA’s
custom-tailored reagent libraries
or our
assay kits, and let us know your specific experimental needs by inquiring.
Interested in gene synthesis?
Inquire with us or submit a project.
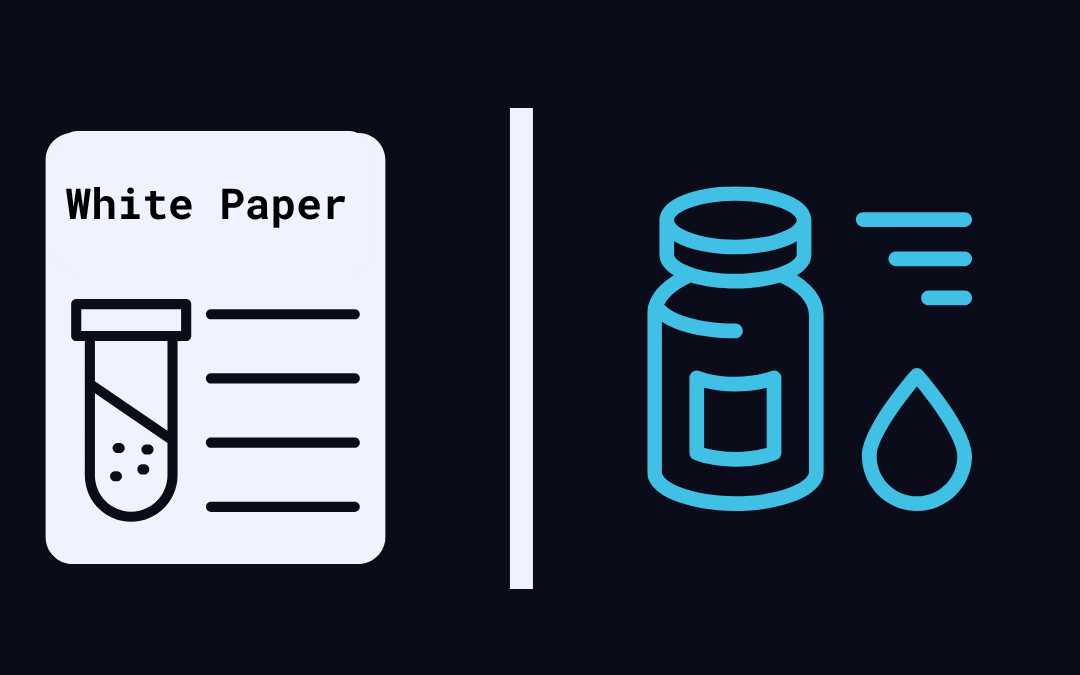
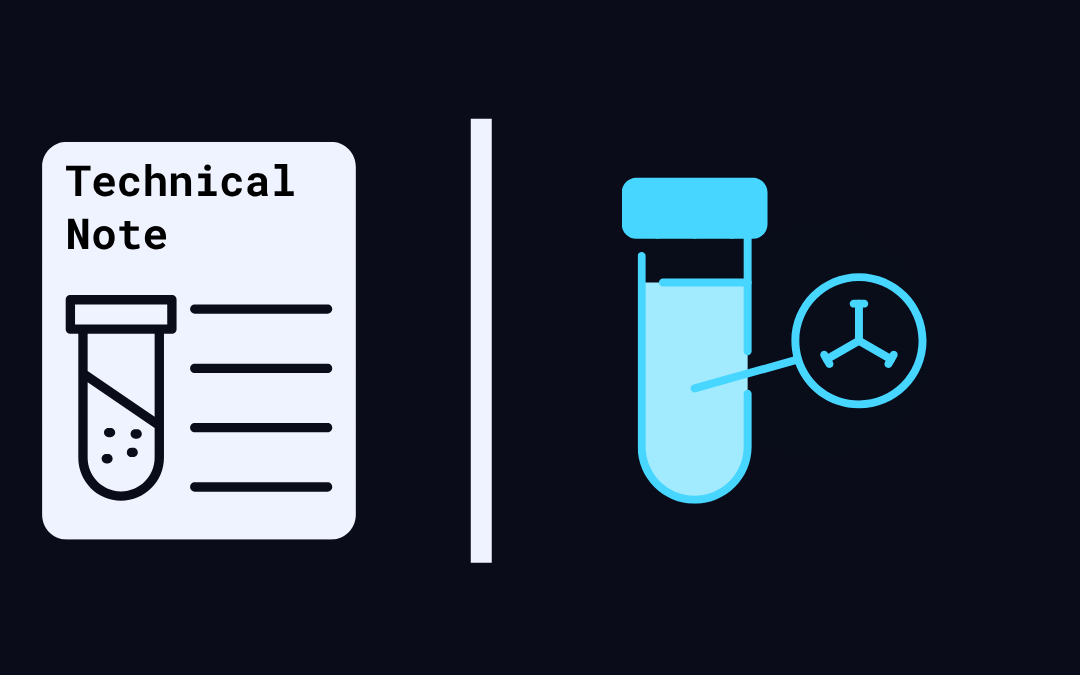